Atomistic electronic models
The workhorse of semiconductor electronic structure theory is k.p envelope theory, which describes the band structure in terms of a few matrix elements that may be empirically determined by fitting to experiments. It is, however, a quasi-continuum theory in which the positions of individual atoms play no role. Recently I have developed with Mats-Erik Pistol a theory of the atomistic limit of k.p theory, which is sensitive to the placement of individual atoms. This opens up an entirely different way of doing nanostructure calculations in which the model is constrained by experimental data, but the structure may be described on the atomistic scale.Spin related phenomena in nanostructures
One of the leading approaches to constructing a quantum computer is to use electrons confined to semiconductor quantum dots. The manipulation and measurement of such confined carriers will be affected and limited by the properties of quantum dots. An understanding of the dynamics of and interactions between electrons in quantum dots is needed to develop a quantum computer, and can only be addressed through detailed numerical computations.The spin of an electron in a quantum dot is effected by electron-phonon interactions, static magnetic fields, polarized light, and intense laser pulses. For dots with more than one electron or hole, Coulomb interactions (especially exchange), give rise to fine structure in the spectrum of electronic states.
Nanowhiskers
A promising new type of quantum dot with better uniformity is the nanowhisker. Since the composition may be varied as the whisker is grown, confinement may be obtained in the direction along the whisker in addition to the transverse direction, leading to 3D confinement. Such structures are under intense experimental investigation, and theoretical results currently lag behind.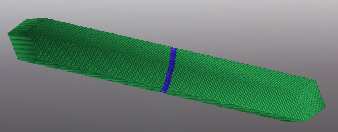